12.08.2013 21:53:00
|
The Hybrid, Electric Vehicle and Fuel-Cell Report Package
NEW YORK, Aug. 12, 2013 /PRNewswire/ -- Reportlinker.com announces that a new market research report is available in its catalogue:
The Hybrid, Electric Vehicle and Fuel-Cell Report Package
http://www.reportlinker.com/p01593897/The-Hybrid-Electric-Vehicle-and-Fuel-Cell-Report-Package.html#utm_source=prnewswire&utm_medium=pr&utm_campaign=NoCategory
This package allows you to buy the Electric Light Vehicle Report, The Hybrid and Plug-in Hybrid Light Vehicle Report, and The Automotive Fuel-Cell Report at a discount.
The Electric Light Vehicle Report
Although today electric vehicle (EV) technology is attracting significant attention and investment, it is certainly not a new sector but the resurgence of one that enjoyed considerable popularity during the latter years of the 19th century and the first decade or two of the 20th. Indeed, before the meteoric rise of the internal combustion engine (ICE) it was electric and steam propulsion that competed for dominance in the motorised transportation sector.
However, the ICE had distinct advantages over the other two technologies, particularly with respect to operating range and convenience: the electric vehicle's batteries frequently required time-consuming recharging and the steam vehicle could not be used before a lengthy warm-up period, and its range was limited by the quantity of water that it could carry. The abundance of cheap oil, the advent of the mass-production assembly line and the development of the self-starter then assured the ICE's advance as the dominant propulsion technology.
This report focuses primarily on vehicles that rely solely on electric propulsion, and also includes a review of 'range-extended' electric vehicles (REEV) that carry an internal combustion engine (ICE) and generator set that is capable of recharging the batteries to enable travel beyond the electric range available from batteries alone. REEVs are predominantly series hybrid vehicles on which the ICE cannot directly power the driving wheels and which feature significant battery capacity.
The Hybrid and Plug-in Hybrid Light Vehicle Report
In a recent interview with IHS SupplierBusiness, Ernie DeVincent, VP Product Development for Getrag commented: "I think it is absolutely inevitable that the penetration of hybrids is going to have to increase. And this will put a great deal of pressure on the economic side of hybrids, particularly battery costs. Because nobody will meet 54 miles per gallon in the US without a substantially higher mix of hybrids than they have today. So hybridisation is going to be a major factor and this puts a lot of pressure on economics".
Hybrid vehicles, although not yet attaining very significant market share, are nevertheless a necessary and growing part of the future powertrain mix. Furthermore, hybridisation has more than just fuel efficiency to contribute in performance terms. The inclusion of hybrid components can deliver desirable customer features such as improved cabin features and HVAC, enhanced launch performance to overcome the weaknesses inherent in downsized engines, high boost turbocharging and optimised transmissions as well as limited electrically driven four-wheel drive (4WD) solutions. Therefore, despite the key aspect of fuel economy improvement, additional value can be delivered to the customer through other technologies associated with hybridisation.
This report looks at the Hybrid and Plug-in Hybrid Light Vehicle sector, which looks at the market drivers, current hybrid architectures and technologies, developing business models and challenges.
The Automotive Fuel Cell Technology Report
OEMs strongly anticipate that from 2015 onwards a quite significant number of fuel cell vehicles will be commercialized with current projections aimed at a few hundred thousand units on a worldwide basis. All OEMs involved will implement road specific production and commercial strategies and, as a consequence, depending on various influencing factors, some commercialisation may occur even earlier than 2015. Indeed, commitments by OEMs to develop hydrogen fuel-cell cars have surged over the past two years. BMW, Toyota, Hyundai, Daimler, Nissan and Honda have all announced plans recently to commercialise the fuel cell drivetrain, in some cases through collaborative agreements in order to spread early technology risk and accomplish economies of scale
In order to ensure a successful market introduction of fuel cell vehicles this market introduction has to be aligned with the build-up of the necessary hydrogen infrastructure. The network should be built up from metropolitan areas via corridors into aerial wide coverage.
About this report
This new report examines the key drivers in this sector and details the main fuel cell types as well as the latest advances in technology. The report goes on to consider fuel cells in the electric powertrain and hydrogen fuel and infrastructure, in particular hydrogen production, hydrogen storage & infrastructure, new chemical approaches and integration with renewable energy.
Finally, the report looks at the development of the automotive fuel cell market with the latest developments from the major automotive manufacturers.
The Electric Light Vehicle Report
INTRODUCTION
Uncertainty and scenarios
Global and local consideration
Grid connectivity, batteries and business models
A brief history of electric vehicles
Electric drive as part of a range of powertrain solutions
MARKET DRIVERS
Fuel economy and CO2 emissions
The United States
The European Union
Japan
China
Other countries
Fuel costs as a driver for grid-connected vehicles
Energy security
Incentives for grid-connected vehicles
The United States
The European Union
China
Japan
South Korea
Canada
India
MARKET CHALLENGES
Recharging infrastructure
Vehicle manufacturers
Charging facilities
Recharging technology companies
Wireless charging technology
Grid capacity Management
Charging Standards
Cost Issues
Range
Recharging time
Resource supplies
Lithium
Rare earth elements
Potential vehicle technology issues
ENABLING TECHNOLOGIES
Batteries and energy storage
Energy and power density
Cycle life
Battery costs
Lithium ion battery construction
Cathodes
Lithium cobalt Oxide - LiCo02
Lithium Manganese Oxide Spinel - LiMn204
Lithium Iron Phosphate - LiFeP04
Lithium (NMC) - Nickel Manganese cobalt - LiNiCo Mn02
Future cathode development
Anode Chemistries
New anode technologies
Graphene based anode technology
CoS2 hollow spheres
Cobalt oxide
Silicon based anode technology
Tin based anode technology
Nano-Tin Carbon Graphene Anodes
Electrolytes and additives
Electrolyte materials
Separators
Cell packaging
Safety circuits
Battery packaging
Manufacturing issues and quality
Chemistry development
Metal-Air batteries
Other battery chemistries
Energy storage membrane
Electric motors
Direct-current (DC) Motors
Asynchronous alternating-current (AC) motors
Synchronous AC motors
Switched reluctance motors
Axial-Flux Motors
In-wheel motors
Electric corner modules
Transmissions
Antonov
BorgWarner
Fallbrook Technologies
Getrag
IAV
Oerlikon Graziano and Vocis
Wrightspeed
Xtrac
Zeroshift
Range extenders
Fuel cell range extenders
Electronic components
Electrically-driven ancillaries
Power steering
Climate control
Regenerative braking
Brakes
Recharging
Electric vehicle supply equipment
Fast charging
Battery exchange
Charging station networks
Inductive charging
EVSE suppliers
New players, relationships and collaborations
Public infrastructure development
Private infrastructure development
Integrated solutions
Integrating the charging infrastructure through IT
MARKET DYNAMICS AND FORECASTS
New markets
Vehicle Market forecasts
Appendix 1 - AVAILABLE ELECTRIC VEHICLES
Electric cars and light commercial vehicles
Range-extended electric vehicles
Appendix 2 - United States incentives for grid-connected vehicles
Appendix 3 - Supplier Profiles
A123
AESC
Aleees
Amberjac
Amperex
Axion Power
Blue Energy Japan
BYD
Continental
Deutsche Accumotive
Dow Kokam
EIG
Exide Technologies
LG Chem
Lithium Energy Japan
SK Innovation
Sumitomo Electric
Valence Techology
Visteon
Yazaki
FIGURES
Figure 1: Well-to-wheel GHG emissions for different powertrain options
Figure 2: Vehicle size and duty cycle aligned to powertrain
Figure 3: Light-duty EV stock forecast under various scenarios
Figure 4: 1900 Lohner-Porsche Rennwagen
Figure 4: GM's EV 1
Figure 6: IEA forecast for alternative powertrains
Figure 7: Well-to-wheel CO2 emissions by powertrain including source considerations
Figure 8: Comparative drivetrain costing per percentage point CO2 reduction
Figure 9: Well-to-wheel powertrain costs relative to conventional
Figure 10: The relative attractiveness of vehicle in Germany 2010
Figure 11: The relative attractiveness of vehicle in China 2010
Figure 12: Different powertrains meet different needs - 2030
Figure 13: Global enacted and proposed fuel economy standards
Figure 14: Lifecycle emissions and fuel use per mile for light gasoline and electric cars
Figure 15: Crude oil (Brent Spot monthly) 1987 to 2013
Figure 16: Comparison of average well-to-wheel CO2 emissions of ICEs with those of EVs powered by the average EU electricity mix
Figure 17: Fuel chain efficiency rates for ICE and EV vehicles
Figure 18: US petroleum product imports 2012
Figure 19: Level 2 charging units from Advanced Energy
Figure 20: SAE J1772 Connectors
Figure 21: SAE J1772 Combined Plug
Figure 22: WPT charging schematic
Figure 23: Evatran's aftermarket available charging system
Figure 24: A floor-mounted induction charge plate
Figure 25: California summer peak loading with unmanaged EV charging scenario
Figure 26: California summer peak loading with work and home EV charging scenario
Figure 27: California summer peak loading with 50% acceptance of differential pricing for EV charging scenario
Figure 28: California summer peak loading with differential pricing for EV charging scenario
Figure 29: Rapidly converging powertrain costs
Figure 30: Powertrain competitiveness in terms of fuel and battery costs
Figure 31: Rapidly converging powertrain costs
Figure 32: Range expectations exceed typical driving distances
Figure 33: Range of EVs launched lags expectations
Figure 34: Energy density improvement over time
Figure 35: European and US consumer expectations of plug-in hybrid range (miles)
Figure 36: EV driving range as a function of ambient temperature
Figure 37: 1990 US driving patterns (miles)
Figure 38: Percentage of daily journeys (km) by country
Figure 39: Charge time expectations by country
Figure 40: Global lithium deposits Lithium Carbonate equivalents)
Figure 41: Lithium demand forecast to 2025
Figure 42: Principal uses of selected rare earth oxides
Figure 43: Projected REE demand at historical growth rates
Figure 44: Inrekor lightweight EV chassis structure
Figure 45: Qualcomm's Halo Wireless EV charging in motion
Figure 46: A graphic representation of vehicle range versus auxiliary load (HVAC) usage
Figure 47: A simple comparison of electrical energy storage systems
Figure 48: The energy density of different fuels
Figure 49: Specific power (W/kg) versus specific energy (Wh/kg)
Figure 50: Cycles by chemistry (deep discharge)
Figure 51: Application cycle requirements
Figure 52: Lithium-ion battery pack cost breakdown
Figure 53: Nominal and usable costs for EV batteries
Figure 54: Patent activity in lithium-ion batteries
Figure 55: Cathode performance compromises
Figure 56: Voltage versus capacity for some electrode materials
Figure 57: Lithium-ion and nanotechnology roadmap
Figure 58: Graphite, soft carbon, hard carbon
Figure 59: Nexeon nano structured silicon anode material
Figure 60: Anode energy density for various anode technologies
Figure 61: Silicon anode dimensional changes
Figure 62: SiNANOde™ silicon graphite composite anode material
Figure 63: LTO anode material
Figure 64: Lithium-ion prismatic battery design
Figure 65: Lithium-ion battery construction
Figure 66: Zinc-Air battery systems
Figure 67: Theoretical maximum energy density for different cell chemistries
Figure 68: Redox battery technology
Figure 69: Technology roadmap for electric traction motors
Figure 70: Typical torque and power comparisons
Figure 71: A schematic of a 6/4 SRM design
Figure 72: An exploded view of a switched reluctance motor's rotor and stator
Figure 73: Axial Flux PM motors
Figure 74: Hiriko Fold pre-production model
Figure 75: Mitsubishi MIEV
Figure 76: Protean Electric's in-wheel electric drive modules
Figure 77: Michelin ActiveWheel
Figure 78: Continental eCorner
Figure 79: Ford Fiesta E-Wheel Drive development vehicle
Figure 80: Optimum EV transmission ratios for each performance criterion
Figure 81: Antonov three-speed EV transmission
Figure 82: BorgWarner 31-03 eGearDrive single-speed transmission
Figure 83: IAV DrivePacEV80
Figure 84: Oelikon Graziano-Vocis two-speed EV transmission
Figure 85: Wrightspeed GTD
Figure 86: Xtrac transmission for the Rolls-Royce 102EX
Figure 87: Chevrolet Volt
Figure 88: Fisker Karma
Figure 89: Lotus range-extender system
Figure 90: Honda FCX Clarity
Figure 91: Continental regenerative braking unit
Figure 92: Mazda regenerative braking using a supercapacitor
Figure 93: Continental spindle-actuated electromechanical brake
Figure 94: A summary of charging locations in the US
Figure 95: A summary of charging locations in the Germany
Figure 96: Different options for grid connection
Figure 97: Better Place battery exchange system
Figure 98: A Schematic of an inductive charging system
Figure 99: GE's WattStation electric vehicle charging station
Figure 101: The vehicle electrification value chain
Figure 100: Changes and opportunities in the automotive value chain
Figure 102: A better place switch station
Figure 103: A Blink charger facility linked to Cisco's Home Energy Controller
Figure 104: 2012 EV sales by country
Figure 105: 2012 EV stock by country
Figure 106: EV stock for selected countries according to EVI
Figure 107: Growth of EV charging facilities in China
Figure 108: Grid-connected vehicle production forecast to 2019 by region
Figure 109: Grid-connected production forecast to 2019 by type
Figure 110: EV and REEV production forecast to 2019 by region
Figure 111: Plugged-in vehicle market forecast – business-as-expected scenario
TABLES
Table 1: 2030 Global market shares of grid-connected vehicles by IHS scenario
Table 2: Global estimates of demand for rare earth oxides 2012
Table 3 Lithium-ion battery cost breakdown
Table 4: Battery cost evolution from 2010 with a CAGR of 14%
Table 5: Four main types of cathode technology in use today (2010)
Table 6: Comparison of typical carbon anode capacities
Table 7: PHEV-EV lithium-ion cell design favoured by various companies (current/ future)
Table 8: Hybrid lithium-ion cell design favoured by various companies (current/ future)
Table 9: Global market for EV charging stations (thousands)
Table 10: Potential roles within the charging infrastructure value chain
Table 11: Comparison of emerging business models
The Hybrid and Plug-in Hybrid Light Vehicle Report
Introduction
• Powertrain choices
• Consumer attitudes
• Development of the Plug-in Hybrid Market
• Cost and value considerations
• PHEV Environmental Performance
Market drivers
• Emissions regulations
The United States
The European Union
Japan
China
Other countries
• Fuel costs
• Criterion emissions
The United States
Japan
Europe
China
Other countries
Hybrid architectures
• Parallel hybrid architecture
• Series hybrid architecture
• Degrees of hybridisation
Full Hybrid
Mild or Assist Hybrids
Plug-hybrids or dual mode
• Aftermarket conversions
Hydraulic hybrid architecture
Flywheel hybrid architecture
Air hybrid
• Vehicle integration
Hybrid technologies
• Higher voltage architecture
• Batteries and energy storage
Energy and power density
Cycle life
Battery costs
Cost breakdown for lithium-ion batteries
• Lithium ion battery construction
Cathodes
Future cathode development
Anode Chemistries
New anode technologies
Electrolytes and additives
Separators
Cell packaging
Safety circuits
• Battery packaging
• Manufacturing issues and quality
• Chemistry development
Metal-Air batteries
Other battery chemistries
• Super-capacitors and ultracapacitors
Energy storage membranes
• Electric motors
Direct-current (DC) Motors
Asynchronous alternating-current (AC) motors
Synchronous AC motors
Switched reluctance motors
Axial-Flux Motors
In-wheel motors.
• Integrated starter-generators (ISG)
Belt-driven alternator-starters (BAS)
Transmissions
One-mode and two-mode hybrids
Getrag
FEV
Fiat Powertrain
IAV
Jatco
ZF Friedrichafen
• Regenerative braking systems and brake blending
• Grid connection and a recharging infrastructure
Vehicle manufacturers
Charging facilities
Recharging technology companies
Wireless charging technology
Developing business models and challenges
• New players, relationships and collaborations
Public infrastructure development
Private infrastructure development
Integrated solutions
Integrating the charging infrastructure through IT
Market development
• Market dynamics and forecasts
• Development of the plug-in hybrid market
New business models for OEMs, grid companies and suppliers
• Market forecasts
North America
Europe
Japan
China
Tables
Table 1: Estimated fuel economy improvement potential and costs relative to 2005
Table 2: US emissions standards for light-duty vehicles, to five years/50,000 miles (g/mile)
Table 3: Japan emissions limits for light gasoline & LPG vehicles (g/km)
Table 4: Japan emissions limits for light diesel vehicles (g/km)
Table 5: Euro 5 emissions limits for light gasoline vehicles (g/km)
Table 6: Euro 5 emissions limits for light diesel vehicles (g/km)
Table 7 Lithium-ion battery cost breakdown
Table 8: Battery cost evolution from 2010 with a CAGR of 14%
Table 9: Four main types of cathode technology in use today (2010)
Table 10: Comparison of typical carbon anode capacities
Table 11: PHEV-EV lithium-ion cell design favoured by various companies (current/ future)
Table 12: Hybrid lithium-ion cell design favoured by various companies (current/ future)
Table 13: Potential roles within the charging infrastructure value chain
Table 14: Comparison of emerging business models
Figures
Figure 1: Roadmap for CO2 reduction
Figure 2: Cost estimates of marginal fuel economy improvement
Figure 3: Carbon dioxide emissions versus cost per percentage fuel reduction
Figure 4: Global plug-in hybrid production forecast
Figure 5: US Annual reduction in GHG production through PHEV adoption in various scenarios
Figure 6: Powertrain electrification 2010 to 2020
Figure 7: PHEV annual costs
Figure 8: Global CO2 (g/km) progress normalised to NEDC test cycle
Figure 9: Fuel economy standards to 2015 for selected countries (US mpg)
Figure 10: WTI crude oil prices (US$ per barrel, monthly average 2010 dollars), 2001 – March 2012
Figure 11: US Regular Gasoline prices $/gallon, January 2011 to June 2013
Figure 12 Emissions standards timetable in selected countries
Figure 13: NOx limits in the EU, Japan and the US, 1995 – 2010 (g/kWh)
Figure 14: PM limits in the EU, Japan and the US, 1995 – 2010 (g/kWh)
Figure 15: Hybrid electric vehicle drive configurations
Figure 16: Charge depletion to charge sustaining transition for PHEV battery packs
Figure 17: An early conversion for the PHEV Prius utilising 15 additional lead-acid batteries
Figure 18: Hydraulic hybrid operation
Figure 19: Torotrak's Flybrid flywheel and IVT system
Figure 20: Hybrid price premium per 100,000 units
Figure 21: Peugeot's air-hybrid architecture
Figure 22: A comparison of air-hybrid architecture efficiency with other types
Figure 23: Additional functions and changes in electrical architecture
Figure 24: Additional functionality requires higher voltages – 48 volts
Figure 25: A simple comparison of electrical energy storage systems
Figure 26: The energy density of different fuels
Figure 27: Specific power (W/kg) versus specific energy (Wh/kg)
Figure 28: Cycles by chemistry (deep discharge)
Figure 29: Application cycle requirements
Figure 30: Lithium-ion battery pack cost breakdown
Figure 31: Patent activity in lithium-ion batteries
Figure 32: Battery costs to OEMs at low volumes
Figure 33: Cathode performance compromises
Figure 34: Voltage versus capacity for some electrode materials
Figure 35: Lithium-ion and nanotechnology roadmap
Figure 36: Graphite, soft carbon, hard carbon
Figure 37: Nexeon nano structured silicon anode material
Figure 38: Anode energy density for various anode technologies
Figure 39: Silicon anode dimensional changes
Figure 40: SiNANOde™ silicon graphite composite anode material
Figure 41: LTO anode material
Figure 42: Lithium-ion prismatic battery design
Figure 43: Lithium-ion battery construction
Figure 44: Zinc-Air battery systems
Figure 45: Theoretical maximum energy density for different cell chemistries
Figure 46: Redox battery technology
Figure 47: Ultracapacitor used to overcome temperature sensitivity to temperature of li-ion battery pack
Figure 48: Ultracapacitor versus lithium-ion energy efficiency
Figure 49: Ultra-capacitor components
Figure 50: Technology roadmap for electric traction motors
Figure 51: Typical torque and power comparisons
Figure 52: A schematic of a 6/4 SRM design
Figure 53: An exploded view of a switched reluctance motor's rotor and stator
Figure 54: Axial Flux PM motors
Figure 55: Mitsubishi MIEV
Figure 56: Protean Electric's in-wheel electric drive modules
Figure 57: Continental's ISAD Unit
Figure 58: Delphi's Belt Alternator Starter
Figure 59: Toyota THS power-split transmission
Figure 60: 2-Mode transmission
Figure 61: Cutaway of a 2-Mode transmission
Figure 62: Getrag's 7DCT300 PowerShift® transmission
Figure 63: Schematic overview of GETRAG 7HDT300 torque-split hybrid
Figure 64: Integrated electric motor cooling options
Figure 65: The advantages of an integrated 48-volt motor solution
Figure 66: FEV's 7H-AMT
Figure 67: Fiat Powertrain compact, lightweight hybrid powertrain concept
Figure 68: Jatco's transmission for parallel hybrid vehicles featuring motor independent drive
Figure 69: Fuel efficiency comparison for ATs
Figure 69: By-wire brake system layout with regeneration
Figure 70: TRW's second generation slip control boost brake technology
Figure 71: Mazda's supercapacitor based regenerative braking system layout
Figure 72: Continental's regenerative braking system layout
Figure 73: Comfortable regeneration requires uncoupling the pedal and quiet and highly dynamic of braking force regulation
Figure 74: Bosch's iBooster unit
Figure 75: Level 2 charging units from Advanced Energy
Figure 76: SAE J1772 Connectors
Figure 77: SAE J1772 Combined Plug
Figure 78: WPT charging schematic
Figure 79: Evatran's aftermarket available charging system
Figure 80: Changes and opportunities in the automotive value chain
Figure 81: The vehicle electrification value chain
Figure 82: A Blink charger facility linked to Cisco's Home Energy Controller
Figure 83: Grid connected vehicles bring changes and opportunities in the value chain
Figure 84: Global plug-in hybrid production forecast to 2020
Figure 85: Global hybrid production forecast to 2020
Figure 86: Global hybrid vehicle production forecast to 2020, by region
Figure 87: Global hybrid vehicle production forecast to 2020, by region
Figure 88: Hybrid sales in the US by model, 1999 - 2012
Figure 89: US hybrid production forecast, 2013 - 2020
Figure 90: European hybrid production forecast, 2013 - 2020
Figure 91:Cumulative Toyota sales
Figure 92: Japanese hybrid vehicle production forecast, 2013 - 2020
Figure 93: Cinese hybrid vehicle production forecast, 2013 - 2020
The Automotive Fuel Cell Technology Report
Introduction
Key drivers
Energy costs and the environment
Fuel Cells and the Automotive Industry
Fuel cell technology
Fuel cell types
Alkaline Fuel Cells (AFC)
Direct Methanol Fuel Cells (DMFC)
Molten Carbonate Fuel Cells (MCFC)
Phosphoric Acid Fuel Cells (PAFC)
Solid Oxide Fuel Cells (SOFC)
Regenerative Fuel Cells (RFC)
Metal Air Fuel Cells (MAFC)
Proton Exchange Membrane Fuel Cells (PEMFC)
Technology progress
Fuel cells in the electric powertrain
FCEV cost development
Hydrogen fuel and infrastructure
Hydrogen production
Hydrogen from coal
Hydrogen production through electrolysis
Hydrogen storage and infrastructure
Hydrogen storage
Hydrogen fuel tanks
Future storage technologies
Liquefied hydrogen
Metal hydrides
Chemical hydrogen storage
Hydrolysis reactions
Hydrogenation/dehydrogenation reactions
New chemical approaches
Carbon nanotube storage
Electrolysis
Integration with renewable energy
Development of the automotive fuel cell market
Daimler
Ford
General Motors
Honda
Hyundai-Kia
Nissan
Toyota
Volkswagen
OEM cooperative agreements
List of Figures
Figure 1: A lightweight hydrogen fuel storage tank [Source: BMW]
Figure 2: A hydrogen fuelling station in California [Source: Hydrogen Association]
Figure 3: Well-to-wheel CO2 emissions by powertrain including source considerations [Source: Eduardo Velasco Orosco, UAEM & GMM]
Figure 4: Well-to-wheel powertrain costs relative to conventional [Source: Eduardo Velasco Orosco, UAEM & GMM]
Figure 5: Technical hurdles overcome in the deployment of FCEVs [Source: EU, McKinsey]
Figure 6: Molten carbonate fuel cell schematic [Source: EERE]
Figure 7: Phosphoric acid fuel cell schematic [Source: EERE]
Figure 8: Solid oxide fuel cell schematic [Source: EERE]
Figure 9: Proton exchange membrane fuel cell schematic [Source: EERE]
Figure 10: Fuel cell stack improvements [Source: GM]
Figure 11: Platinum loadings for PEM fuel cells [Source: US DOE]
Figure 12: Schematic representation of the functionality of a fuel cell [Source: PEMAS]
Figure 13: System schematics for 2008 and 2009 fuel cell system [Source: US DOE]
Figure 14: System schematics for 2010 and 2015 fuel cell systems [Source: US DOE]
Figure 15: Net system cost versus annual production rate [Source: US DOE]
Figure 16: Coal gasification process [Source: US DOE]
Figure 17: Sulphur Iodine cycle for H2 production [Source: Hydrogen Energy]
Figure 18: Conventional electrolysis for H2 production [Source: Hydrogen Energy]
Figure 19: Commercially available solutions for on-board hydrogen storage [Source: US DOE]
Figure 20: BMW's Cryo-compressed hydrogen storage system [Source: BMW]
Figure 21: Hydrogen mass and cost comparison of compressed (700 bar) and cryo-compressed (350 bar) storage [Source: BMW]
Figure 22: a schematic of MOF-74 metal organic framework [Source: NIST]
Figure 23: Mercedes-Benz F125 fuel cell plug-in hybrid [Source: Daimler]
Figure 24: Molecular hydrogen storage in light element compounds [Source: US DOE]
Figure 25: Schematics of nanotube structures [Source: Nanotechnologies]
Figure 26: Schematic of a three-dimensional nanotube matrix [Source: RSC]
Figure 28: European national initiatives for hydrogen infrastructure [Source: NOW]
Figure 28: Publically accessible hydrogen refuelling stations – Germany [Source: NOW]
Figure 29: Planned development of hydrogen refuelling infrastructure in Germany [Source: NOW]
Figure 30: Hydrogen refuelling site Oslo using two Hydrogenics electrlysers [Source: Hydrogenics]
Figure 31: Honda's prototype solar hydrogen refuelling station in Los Angeles [Source: Honda]
Figure 27: ITM Power's HFuel transportable hydrogen refuelling station [Source: ITM Power]
Figure 28: OMV hydrogen refuelling site Stuttgart [Source: Daimler]
Figure 29: Percentage energy generation from renewable sources [Source: Geocurrents]
Figure 31: London hydrogen fuelling station used by fuel cell buses [Source: Air Products]
Figure 32: A schematic for an 'artificial leaf' [Source: Science Now]
Figure 37: FECV and BEV contributions to CO2 reductions [Source: Various]
Figure 38: Mercedes-Benz B-Class F-Cell [Source: Daimler]
Figure 39: Daimler's F125!fuel cell hybrid concept [Source: Daimler]
Figure 40: Fuel cell Chevrolet Equinox [Source: GM]
Figure 42: Honda's Clarity fuel cell car [Source: Honda]
Figure 41: Schematic of the Honda Clarity [Source: Honda]
Figure 43: The first production model of Hyundai's ix35 fuel cell vehicle [Source: Hyundai-Kia]
Figure 43: Toyota's FCV-R fuel cell concept car [Source: Toyota]
Figure 45: OEM forecast fuel cell vehicle production [Source: IHS]
Figure 46: Geographic forecast fuel cell vehicle production [Source: IHS]
List of Tables
Table 1: A comparison of fuel cell technologies [Source: US DOE]
Table 2: Technical targets for automotive applications [Source: US DOE]
Table 3: A summary of system costs for 2010 and 2015 technologies at various manufacturing rates [Source: US DOE]
Table 4: US hydrogen refuelling stations 2012 [Source: www.fuelcells.org]
To order this report:
: The Hybrid, Electric Vehicle and Fuel-Cell Report Package
__________________________
Contact Clare: clare@reportlinker.com
US:(339) 368 6001
Intl:+1 339 368 6001
SOURCE Reportlinker
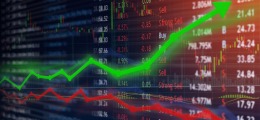
Wenn Sie mehr über das Thema Aktien erfahren wollen, finden Sie in unserem Ratgeber viele interessante Artikel dazu!
Jetzt informieren!